Exploring the Basics of Indium Phosphide (InP)
The seemingly humble binary semiconductor, Indium phosphide (InP), has been stirring a sense of intrigue within the realm of optoelectronics due to its unique attributes. This mysterious material is no ordinary player – it boasts some truly remarkable optical and electron characteristics that position it as an attractive contender in not just one but several applications; think solar cells or photovoltaic devices. The speed at which its electrons move surpasses that of other materials like silicon or gallium arsenide, allowing InP to facilitate quicker signal processing speeds.
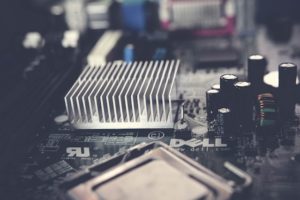
But there’s more – the direct bandgap InP possesses gifts it with superior light emission capabilities, a crucial advantage when we delve into all things optoelectronic.
Venturing further into this captivating world reveals recent advancements in thin film technology which have broadened horizons for potential uses of InP beyond conventional bulk structures. As if by magic, thin films of indium phosphide can be crafted using various techniques that yield stringent control over thickness and uniformity. These methods also permit the birth of intricate nanostructures such as quantum dots nestled within the matrix of these thin-film formations. Blessed with properties distinct from their bulk counterparts due to effects known as quantum confinement effects, these nanostructures are opening doors to exciting new dimensions in device design and function.
Characterization processes emerge as a vital component in decoding these newly synthesized marvels based on InP-based photovoltaic devices; they serve as guides illuminating strengths and weaknesses, thus aiding optimization endeavors for enhanced performance efficiency.
An important caveat remains though: while Indium Phosphide holds significant promise for future solar cell development – courtesy its inherent qualities combined with advanced fabrication methodologies available today – there are still hurdles looming ahead related to cost-effective large-scale production among others that need conquering before widespread embracement can transpire.
Understanding Thin Film and Its Role in Solar Cells
The realm of semiconductor industry has been largely captivated by the synthesis of indium phosphide (InP), mainly due to its unrivaled optical and electron characteristics. When fashioned into thin films, this compound proves itself a superior performer in optoelectronic devices such as solar cells; a strength that can be attributed primarily to InP’s direct bandgap that harmoniously aligns with the solar spectrum. This alignment facilitates an efficient absorption and conversion process of light energy. To further augment their photovoltaic efficiency, these thin films are interspersed with quantum dot structures.
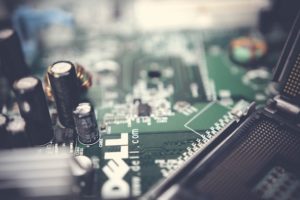
A significant factor in synthesizing high-caliber InP-based solar cells revolves around the meticulous selection and preparation procedures for substrates—silicon being a prevalent choice owing to its plentiful availability and well-defined processing methods. It is critical however, to acknowledge that silicon surfaces are prone to forming an oxide layer which could potentially compromise device performance via interface defects or obstructive charge transport. As means of countering this issue, approaches such as surface passivation or oxide elimination have been put into practice before depositing the InP thin film onto the silicon substrate.
Thin film technology holds more than just one crucial role—it stands paramount not only in optimally harnessing sunlight but also significantly reducing material usage when juxtaposed against traditional wafer-based technologies—an indispensable aspect from both economic and environmental standpoints. For instance, typical thicknesses for semiconductor layers within thin-film solar cells oscillate between several nanometers (nm) up till roughly 2 micrometers (µm). This renders them considerably thinner compared to conventional wafers boasting hundreds of micrometers in thickness.
Optical and Electron Properties of InP
In the realm of optoelectronic applications, Indium Phosphide (InP) has emerged as a desirable choice due to its unique optical and electron attributes. This compound semiconductor material is characterized by a band gap of 1.35 eV at room temperature, enabling it to efficiently interact with light in the infrared spectrum – absorbing and emitting effectively. Its aptitude for being used in lasers and photodetectors stems from this property.
Moreover, InP stands out by displaying high current density; thus making it suitable for electronic devices that demand high speed – particularly those functioning at microwave frequencies.
Adding another layer of intrigue is the nanostructure of InP which plays an instrumental role in enhancing its performance in optoelectronics. Detailed studies using spectroscopic techniques have unveiled that through meticulous manipulation of InP’s nanostructure, one can significantly augment its quantum efficiency. For instance, when these materials are formulated into nanowires (InP NWS), they demonstrate heightened absorption characteristics across diverse wavelengths owing to their expanded surface area compared to bulk materials.
Further examinations on InP divulge that its fill factor – indicative of maximum potential power output from a solar cell – ranks among the highest recorded for any semiconductor material utilized in photovoltaic operations. This hints towards the prospect that solar cells composed from InP might offer more power per unit area than units based on alternative materials like GaAs.
A peculiar feature inherited within photons less energized than the bandgap energy allows them passage without absorption, while those possessing higher energies contribute energy equivalent only up to the bandgap energy towards current generation upon absorption; thereby amplifying its prospective application scope within multi-junction solar cells where each stratum absorbs light within designated wavelength boundaries.
Harnessing Quantum Dot and Nanostructure in Solar Cell Development
The realm of quantum dots and nanostructures is increasingly being illuminated for their conceivable potential in the formation of solar cells. Their epitaxial growth, a process akin to meticulously stacking delicate layers, provides supreme control over their physical properties – a feature that significantly enhances light absorption and boosts photovoltaic performance. Remarkably, they show outstanding electrical traits even at room temperatures, establishing themselves as optimal contenders for application across various optoelectronic devices.
Specifically standing out in this context is indium phosphide (InP), known for its distinctive characteristics. Quantum dots made from InP have demonstrated an impressive ability to amplify short circuit current density when infused into the absorber stratum of photovoltaic mechanisms. This can be largely credited to InP’s high-temperature stability which preserves device function under severe sun exposure conditions. In addition to these merits, it’s significant to mention that phosphorus presence contributes towards diminishing open circuit voltage losses usually linked with other materials employed in analogous applications.
The role played by the fabrication technique cannot be understated when it comes to extracting optimum performance from these quantum dot-based solar cells. Conventional etching strategies often jeopardize or modify the III-V semiconductor materials involved; however, recent breakthroughs propose more accurate methods that mitigate such hazards while boosting overall cell productivity. It would also be worth mentioning here that fine-tuning parameters like bandgap energy can further enhance light absorption rates thus upgrading total photovoltaic yield.
Optoelectronic Devices and Their Photovoltaic Applications
Optoelectronic contrivances are steadily drawing the scientific eye due to their potential in harnessing solar energy. These unique devices astound with dual properties – optical and electrical, which can be deftly manipulated for efficient transformation of power. The creation process is complex, involving material growth on InP substrates through a range of deposition techniques. A tool often called upon during this intricate procedure is the scanning electron microscope; a watchdog ensuring precise regulation of both crystal structure and dimensions.
Diving deeper into these optoelectronics reveals many materials with direct band gap nature, enabling them to absorb light effectively – an attribute that renders them perfect candidates for solar cell applications. Among these promising materials lies one standout: the quantum well structure, born from cutting-edge nanofabrication techniques. This petite nanostructure boasts high external quantum efficiency thanks to its knack for corralling electrons within minute volumes thereby encouraging efficient absorption and emission processes. Thus, it’s no surprise that transmission electron microscopy studies play a pivotal role in investigating these structures as they provide crucial perspectives into their minutiae.
Take for instance when an electron beam partners up with a transmission electron microscope – together they disclose comprehensive information about atomic arrangements inside such structures while unmasking any flaws or inconsistencies that could potentially hamper performance under photovoltaic conditions. As if not enough, researchers have even discovered ways to tweak parameters like temperature and pressure mid-growth on InP substrates allowing them to custom-fit the optoelectronic characteristics of these materials without risking structural soundness or operational steadiness.
Synthesis of Indium Phosphide: Methods and Characterization
With a broad band gap and high electron mobility, Indium Phosphide (InP), a III-V semiconductor, has become a subject of immense interest in the realm of optoelectronics. The InP wafer is typically birthed from methods such as metal-organic chemical vapor deposition (MOCVD). This technique is often harnessed to create p-type InP, exploiting properties like quantum confinement and elevated electron-hole recombination rate – facets that make this material ideal for various applications.
Post-synthesis characterization follows suit, involving an array of techniques designed to decipher the physical and electronic attributes of the newly synthesized substance. Electron diffraction stands out as one such method; it serves as a potent instrument used for probing structural intricacies at the atomic level. It divulges indispensable insights into aspects such as lattice constants, crystalline orientation, defect structures among others – all cardinal factors in determining performance traits of devices made from these semiconductors.
Further examination can be carried out via methods like Electron Beam Lithography (EBL). EBL permits meticulous patterning on semiconductor surfaces thus facilitating creation of minute scale features or nanostructures on their surface. These include semiconductor quantum dots or even nanowires which are subsequently scrutinized under assorted conditions to fathom their conduct under fluctuating operational circumstances. The information harvested through these characterization methods aids researchers in refining device fabrication procedures ensuring peak efficiency while upholding durability and reliability standards established by industry norms.
The Role of Oxide and Substrate in Silicon-Based Solar Cells
In the realm of silicon-based solar cells, there’s a predominant reliance on oxide and substrate for their performance. A crucial element playing a significant part in this process is the formation of InP (Indium Phosphide), particularly within thin layer structures. The influence it holds over both electrical and optical properties is substantial.
To achieve peak performance, fabrication processes need to be meticulously precise – enter reactive ion etching, used to fashion an orderly array of InP nanowires. These are typically deposited at room temperature which affords superior control over their dimensions and orientation.
Probing deeper into these structures necessitates specific characterization methods such as field emission scanning electron microscopy (FESEM) and Fourier transform infrared spectroscopy (FTIR). FESEM provides high-resolution imagery that allows researchers an intimate view into the morphology and distribution of semiconductor quantum dots inside the device.
Then there’s FTIR – instrumental in identifying chemical bonds present after deposition or processing stages have occurred. It’s important to underline that both techniques play pivotal roles in decoding how factors affect optical and electrical properties.
This innovative evolution has expanded possible applications for silicon-based solar cells beyond conventional use cases. For example, they’re showing encouraging results when considered for space applications due to their resilience against harsh environmental conditions along with remarkable efficiency rates.
Devices rooted in this technology continue being scrutinized extensively thanks to their ability to marry top-tier performance with cost-effectiveness—a blend potent enough potentially revolutionize renewable energy solutions across various sectors worldwide.
Band Gap and Current Density: Vital Aspects in Solar Cells
In the realm of InP device performance, the crafting or synthesis of indium phosphide (InP) is a pivotal determinant. The process, intricate in its execution, necessitates several stages including depositing white phosphorus and indium initially. Following this comes selective deposition of InP to ensure top-notch material output. Moreover, sophisticated methods like selected area electron diffraction are employed for thorough characterization which aids in deciphering the structural attributes and quality of synthesized InP.
The introduction of nanostructures such as those based on InP – quantum dots and nanowires- has initiated a transformation wave in solar cell development. These entities have demonstrated exceptional optical properties enabling them to cover a larger spectrum region compared to their traditional counterparts used in solar cells. They also showcase steadfast performance even amidst defects or impurities due to their distinct electronic structure.
Moving forward towards bandgap engineering – an essential aspect that dramatically influences solar cell efficiency stands tall. By tweaking this property using diverse techniques like alloying or doping with other elements can customize absorption traits per specific requisites. For instance, merging the forces of InP and GaP nanowires could potentially enhance light absorption across multiple spectral regions thereby boosting overall photovoltaic performance while maintaining optimal current density levels within these devices.
Comments are closed.