Understanding Gallium Arsenide: A Detailed Look at its Properties and Applications
In the realm of electronics, Gallium Arsenide (GaAs) – a blend of gallium and arsenic – has emerged as a noteworthy player. Unlike its silicon counterparts, this semiconductor material is noted for its high electron mobility and direct bandgap properties. This makes it an exceptional choice for high-frequency applications and optoelectronic devices such as lasers and solar cells respectively.
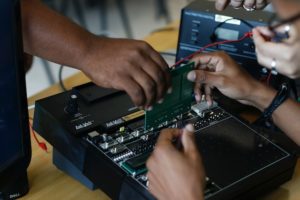
Further distinguishing itself from the crowd, GaAs wafers exhibit less noise in electronic devices which enhances their reliability factor significantly. Hence, despite Silicon’s dominance owing to cost-effectiveness and well-established manufacturing infrastructure, GaAs secures a unique foothold within specific sectors.
Its inherent radiation resistance property teamed with superior performance at heightened temperatures gives GaAs an edge in aerospace applications where these traits are crucial. The advent of any semiconductor device requires wafer fabrication—an area where GaAs stands tall over Silicon once again.
The production process necessitates growing single-crystal ingots by meticulously adding layers of gallium arsenide onto a substrate wafer under regulated conditions—a technique often termed epitaxial growth. This method gifts manufacturers enhanced control over layer thickness and uniformity that consequently boosts performance capabilities in resulting GAAS devices.
This exclusive amalgamation of physical attributes continues to propel research into new potential avenues for Gallium Arsenide within our increasingly technologically advanced society—proving yet again that what sets you apart can sometimes be your greatest strength.
Production and Manufacturing Process of Gallium Arsenide Wafers
The intricate artistry of producing GaAs wafers begins with the inception of an ultra-pure, singular crystal substrate. The single-crystal GaAs substrate is nurtured into existence through techniques such as Liquid Encapsulated Czochralski (LEC) or Vertical Gradient Freeze (VGF), meticulously crafted to minimize any imperfections and ensure a high rate of electron mobility. This pivotal stage sets the foundation for fabricating devices like transistors and diodes which demand high conductivity coupled with low defect density.

Upon completion of this growth phase, our wafer enters the realm of epitaxial deposition – a method that sculpts thin layers onto the substrate by assembling atoms layer by layer. In this process, epitaxy guarantees flawless alignment between each atom across both layers, resulting in an impeccably pure crystal structure boasting superior electrical properties when juxtaposed against silicon-based substrates. It’s at this juncture that doping transpires; specific impurities are deliberately woven into the lattice framework to tweak its conductive features depending on whether n-type or p-type semiconductors are sought after for integrated circuit applications.
Beyond their role in integrated circuits, GaAs wafers have found widespread utility within solar cells due to their wide band gap attributes allowing them superior efficiency in translating sunlight into electricity. Coupled with semi-insulating properties when left undoped they emerge as prime candidates for specific electronic devices including LEDs and laser diodes. Despite carrying a higher price tag than silicon equivalents, GaAs has etched its own territory within semiconductor market largely thanks to its elevated electron mobility and enhanced ability resist heat compared to silicon-based gadgets – factors propelling it towards becoming a preferred choice within semiconductor industry.
The Role of Arsenic and Gallium in Forming GaAs Semiconductor Material
The realm of optoelectronic and microwave devices has found a formidable ally in the form of Gallium Arsenide (GaAs), a compound semiconductor that boasts unique material properties. The birth of this potent entity requires an intricate dance between gallium and arsenic, culminating in the creation of complex single-crystal structures. These grand lattice structures are teeming with high electron mobility, a trait that beckons laser diodes operating within the infrared spectrum.
When producing GaAs, one must not overlook the epitaxial growth method – an approach revered for its ability to deposit atom layers onto crystalline substrates while honoring their orientation. This careful procedure gives rise to impressively pure single crystal layers of GaAs but is fraught with challenges such as ion implantation issues and arsenic loss during manufacturing. Nevertheless, relentless research continues unabated to overcome these stumbling blocks and enhance traits like thermal conductivity.
What also sets GaAs apart from others is its extraordinary valence structure which arms it with superior dielectric properties when compared to silicon-based semiconductors – another feather in its cap. Unlike silicon’s restrictive nature where electrons can only exist at specific energy levels or bands within its lattice structure, GaAs offers greater freedom by allowing electrons across different energy bands. This flexibility makes it indispensable for various electronic devices including photovoltaic cells and light-emitting diodes (LEDs).
These tantalizing features have sparked global intrigue among scientists who are on an unceasing mission: optimize Gallium Arsenide’s potential through refining processing techniques and deepening understanding about this enigmatic yet promising material characteristics.
A Comparative Study: GaAs and Silicon in the Semiconductor Market
For an extensive stretch of time, the semiconductor marketplace has been in the iron grip of silicon-based devices. Their reliability and cost-effectiveness have made them almost omnipresent, from their integration into circuits that power our computers and smartphones to their indispensable role in the renewable energy generation via solar panels. The fabrication process necessitates the use of thin slices known as silicon wafers, which are gleaned from single-crystal chunks conspicuously referred to as boules.
However, a new contender has burst onto this scene with perplexing potential: gallium arsenide (GaAs). This compound arises when gallium – also familiarly dubbed ‘gallium is called,’ amalgamates with arsenic atoms under certain environs using a technique intriguingly named molecular beam epitaxy. GaAs’s characteristics render it alluring for specific applications; one such enticing feature is its higher electron mobility enabling devices crafted out of gaas to function at frequencies beyond those achievable by conventional silicon technology. While promising, these properties come with complications during production processes compared with standard silicon-based semiconductors—constructing an epitaxial GaAs layer on a single-crystal GaAs substrate demands advanced manufacturing techniques like ion implantation or chemical vapor deposition.
Transitioning from trusted silicon technologies towards unknown territory that is GaAS can be considered quite risky given unproven aspects related to contact reliability over time—a predicament that adds complexity to the promise held by this material. Moreover, while semi-insulating GaAS offers superior speed and frequency response compared to another competitor – Gallium Nitride(GaN), its performance pales when you factor in thermal conductivity where Silicon significantly surpasses both materials’ capabilities. Hence while there’s tantalizing growth potential within integrated circuit technologies employing gaas including RF communication systems or optoelectronic devices; more research needs addressing key issues surrounding dissociation of gaas during device operation before widespread acceptance becomes viable.
The Implication of GaAs in Integrated Circuit Technologies
In the realm of semiconductor manufacturing, silicon-based technologies have long held sway. Yet, a shift is detectable, an increasing awareness of the potential inherent in gallium arsenide (GaAs) technology. This compound boasts unique characteristics that differentiate it from existing contestants in the semiconductor field like silicon and silicon carbide – all due to its unique composition of two elements: gallium and arsenic.
By virtue of their atomic structure with three valence electrons for gallium and five for arsenic, they form a covalent bond culminating in direct band gap material teeming with superb optoelectronic properties. GaAs excels particularly where high-frequency applications are involved such as weak-signal amplification thanks to its superior electron mobility compared to silicon.
Unlike its popular counterpart – Silicon – GaAs showcases low thermal conductivity which can result in considerable thermal gradients across non-isothermal surfaces at sub-micron length scales. Such characteristic demands comprehensive understanding regarding heat generation within these devices alongside accurate models predicting both heat generation and temperature for effective design application.
Yet this promising tech has been met with caution by organizations unwilling to abandon or jeopardize their established processes revolving around silicon semiconductors without compelling reasons or enticing rewards. The shift would imply massive retooling expenses along with uncertainty related to yield rates during initial production ramp-up phases.
Despite these challenges linked with GaAs technology adaptation, there is no denying its appealing attributes when applied specifically towards certain semiconductor tasks where traditional materials may not perform effectively or efficiently; thus more establishments find themselves drawn towards this path conscientiously exploring possibilities it offers.
Comments are closed.